Research
Explaining the properties of solids from a first-principle, atomistic perspective is a tremendously difficult problem due to the need of solving the many-particle Schrödinger equation. Only with the conception of density functional theory (DFT) and its local density approximation (LDA) numerical computations for real materials became feasible. The successes of DFT in explaining the electronic properties of many elements and compounds, and its applicability to many quantum-chemical problems, are extraordinarily impressive. Nonetheless, the effective single particle language of Kohn-Sham DFT, is not sufficient for an analysis of the excitation spectrum when many-body effects exceed a certain strength. Hence, the family so-called strongly correlated electron systems turned out to be beyond the possibilities of the DFT description. As a consequence, the treatment of such systems has been limited to model-type approaches. Experimentally, however, specifically such correlated systems show a great potential for unusual phenomena. Mott insulators, heavy-fermion rare-earth compounds with unconventional superconductivity, colossal magneto-resistance in manganites and, probably the most intriguing phenomenon of all, high temperature superconductivity in cuprates.
In the last decade a part of the experimental community has started to work on correlated systems which can be "artificially" constructed on an atomic level. The goal of the Max Planck Research Group "Electronic Structure of Correlated Materials" is to provide theoretical support for interpretation of experiments and, even more importantly, for exploring novel materials. Our tools include the merger of density functional theory (DFT) with many body methods like dynamical mean field theory (DMFT) and extensions like extended DMFT (EDMFT) or even GW+EDMFT as well as configuration interaction (CI) cluster calculations for modern x-ray spectroscopies. Parameter free calculations are possible with calculation of screened Coulomb potentials by constrained random phase approximation (cRPA) which guide us to a realistic low energy effective Hamiltonian of a given material. Specific projects include:
Correlated adatoms on semiconductors
In 1974 P. W. Anderson and E. Tosatti suggested to study correlation effects in surface states of a quasi two-dimensional lattice of group IV atoms (C, Si, Sn, Pb) deposited on a silicon or germanium surface X:Si(111) and X:Ge(111). Only recently, however, experimental and theoretical technology have reached the necessary level of sophistication to synthesize / simulate these compounds. Different from common correlated materials like transition metal oxides the low energy effective Hamiltonian is not of the Mott-Hubbard type but has to include also non-local interaction terms [1]. Only with the help of recently developed extensions of dynamical mean-field theory: extended DMFT (eDMFT) and eDMFT+GW such non-local interactions were identified as key to the unification of the X:Si(111) family in a rich phase diagram (Fig. 1) [2].
Moreover, with the help of the calculated charge-charge correlation function (Fig. 2) a long standing controversy of Sn:Si(111) can be resolved: Due to its position in the phase diagram right next to the phase transition between Mott insulator and CO insulator and the resulting fluctuations (and even phase coexistence) the experimental result crucially depends on the time/frequency range of the measured observable: "Slow" STM measurements do not detect any difference to "pure" Mott materials of the family (like C:Si(111) and SiC(111)) and only higher energy spectroscopies like core-level photoemission are able to capture the described charge fluctuations.
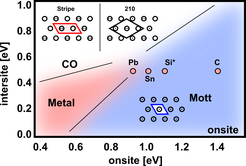

Fig. 2: Charge-charge correlation function of Sn:Si(111) plotted in real space at t=0. Short lived, finite size fluctuations are detected only by sufficiently "quick" experiments as precursors of the charge ordered phase.
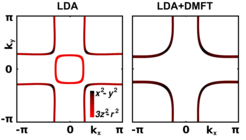
Oxide heterostructures
Driven by trends seen in the electronic structure of high-Tc cuprate superconductors [3] and speculations about creating a cuprate analog electronic structure in rare earth nickelate heterostructures [4], we predicted some time ago that rare earth nickelate based heterostructures can be engineered to have a cuprate-like Fermi surface (Fig. 3) and should be considered as promising candidates for high-Tc superconductivity [5]. While experimentally there has been significant progress in "orbital engineering" of nickelate superstructures, theory remains to this date inconclusive and struggles in particular with the treatment of oxygen degrees of freedom. We find (as a general result for many transition metal oxides like V2O3, NiO, and high-Tc cuprates) that a "naive" inclusion of oxygen 2p states as uncorrelated background is rather dangerous and leads to questionable ground state configurations (dp-models for cuprates treated this way, e.g., will not have insulating ground states unless 2p-states are removed "by hand" [6]).
In collaboration with experimental groups, in particular the Minerva Research Group "X-ray Spectroscopy of Oxide Heterostructures" (headed by Eva Benckiser) we will tackle these challenges ranging from model- to specific material-studies.