Nature of charge gap and excitonic transition in Ta2NiSe5 revealed by scanning tunneling microscopy and thermal transport
A pair of experiments sheds new light on the excitonic insulating properties of the hotly debated compound Ta2NiSe5
Superconductivity, the lossless flow of electrical current, is explained by the somewhat counterintuitive notion that electrons with mutually repulsive Coulomb interactions can nevertheless form bound pairs. This phenomenon is observed in a wide range of metals at low temperatures. Ironically, the spontaneous condensation of electron-hole pairs with mutually attractive Coulomb interactions, i.e., an excitonic insulator, has proven to be much more elusive. First proposed in 1967 [1], the excitonic insulator remains challenging to materialize in a bulk crystal and distinguish from an ordinary insulator.
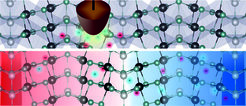
The layered ternary chalcogenide Ta2NiSe5 is arguably the leading candidate for an excitonic insulator in a bulk crystal. It exhibits a transition from a zero-gap semiconductor to an insulator below Tc ≈ 326 K. First synthesized in the 1980s [2], Ta2NiSe5 soon fell by the wayside, because it lacked a charge-density-wave state that was highly sought for during those days. In 2009, interest in Ta2NiSe5 was revived by photoemission experiments revealing the flattening of the top of the valence band below Tc, a possible signature of an excitonic insulator gap [3]. Since then, mounting evidence for an excitonic insulator has accrued, including a gap that is similar in value to the expected exciton binding energy and a Tc that traces out a dome shape with pressure and chemical substitution [4].
Not everyone is convinced. The electronic transition that takes place at Tc ≈ 326 K is accompanied by a structural transition from an orthorhombic to monoclinic unit cell. The structural distortion allows the Ta 5d and Ni 3d orbitals to hybridize, resulting in a single-particle band gap. It has become increasingly clear that two phenomena – a many-body gap due to an excitonic insulator, and a single-particle gap due to band hybridization – must be inevitably intertwined in Ta2NiSe5 [5]. The question now is how "excitonic insulating" is Ta2NiSe5, and how such properties can be revealed.
In one experiment by He et al. [6], the authors find that by positioning the tip of a cryogenic scanning tunneling microscope within a few angstroms of the Ta2NiSe5 surface, the insulating phase collapses abruptly into a zero-gap semiconductor. The proximity of the tip induces a local electric field and introduces additional surface charges. Whereas a band insulator would remain stable to charge doping, an excitonic insulator, with equal number of electrons and holes, would be rapidly destabilized by the addition of excess charges. The observation of a tip-induced gap collapse in Ta2NiSe5 thus points to the predominant many-body character of its charge gap.
In another experiment by Zhang et al. [7], the authors employ thermal transport to elucidate the distinct behaviors of charge and lattice degrees of freedom across Tc. Thermal transport is particularly well suited because it can detect all mobile quasiparticles, including electrons and holes with zero total charge and phonons. The authors observe a striking anisotropy: When the heat current flows parallel to the one-dimensional chains of Ta2NiSe5, the thermal conductivity shows an abrupt decrease below Tc due to electrons and holes, indicative of an excitonic transition. When the heat current flow perpendicular to the chains, the thermal conductivity shows a V-shaped suppression due to phonons that persists far above Tc, indicative of phonon softening, likely coupled to excitonic fluctuations.
References:
[1] D. Jérome et al., Phys. Rev. 158, 462 (1967).
[2] S. Sunshine and J. A. Ibers, Inorg. Chem. 24, 3611-3614 (1985); F. J. Di Salvo et al., J. Less Common Metals 116, 51-61 (1986).
[3] Y. Wakisaka et al., Phys. Rev. Lett. 103, 026402 (2009).
[4] Y. F. Lu et al., Nat. Commun. 8, 14408 (2017).
[5] G. Mazza et al., Phys. Rev. Lett. 124, 197601 (2020).
[6] Q. He et al., Phys. Rev. Research 3, L032074 (2021).
[7] Y.-S. Zhang et al., Phys. Rev. B 104, L121201 (2021).