Measuring Electron Oscillations in a Quantum Nanodevice
The pursuit of realizing light-wave electronics, where one is able to drive, manipulate, and store electronic oscillations in solids by using light has the key perquisite of being able to directly measure electron oscillations. Nonlinear optical techniques can measure the consequence of the ultrafast electron oscillations in solids, but cannot unambiguously measure them directly in time. Researchers from Max Planck Institute for Solid State Research (MPI-FKF, Stuttgart) and University of Stuttgart have now developed an innovative self-referencing method to directly measure electron oscillations in a quantum nanodevice.
Interaction of light with matter inevitably leads to oscillation of electrons in matter at the frequency of the light, which is very high, ≈0.3 PHz (one PHz = 1015 Hz). This frequency of electron oscillations is nearly a million times higher compared to the fastest electron oscillations that can be induced and measured in conventional electronics, which is nearly one GHz (1 GHz = 109 Hz). Scientists have been stimulated by the possibility of driving electrons in solids (for example, in integrated chips) by using light pulses and using it as a means to achieve electronics, where one can perform logic operations with electron oscillations and later store them to perform computing.
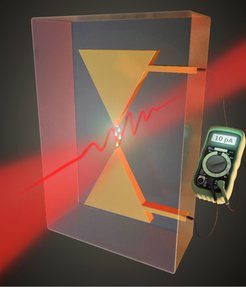
One of the key steps in realizing light-wave electronics is being able to drive and directly measure electron oscillations in solids. Nonlinear optical techniques can indirectly measure the consequence of light-driven electron oscillations by observing changes in the property of the driving light (for example, reflectivity). Now reporting in Nature Communications, researchers from MPI-FKF and University of Stuttgart have developed the technique of optical homodyne beating to measure electron oscillations in an on-chip nanodevice. In optical homodyne beating, researchers interfere electron oscillations driven by two light pulses, whose center wavelengths are slightly different from each other. This approach works as a self-referencing technique, where electron oscillations driven by one light pulse measures the oscillations driven by the second light pulse. The on-chip nanodevice used in the work comprised of gold bowtie nanoantenna with junction gaps of only a few nanometers, as pictorially depicted in the image.
The self-referencing technique also allows to measure nonlinear electron oscillations in the on-chip nanodevice, which can be induced in the device at higher powers of the driving light.
The capability to coherently trigger, measure and control electron oscillations, opens new prospects for understanding strong light-matter interaction in solids directly in the time domain. Additionally, it paves the way towards on-chip light-wave electronics at petahertz (PHz) switching and read-out frequency.