Magnetic monopoles in spin ice
Inseparable, that is what the north and the south pole of a magnet usually are. In some solids, however, they can move independently and thus become magnetic monopoles.
Inseparable, that is what the north and the south pole of a magnet usually are. In some solids, however, they can move independently and thus become magnetic monopoles.
Text: Christian Meier
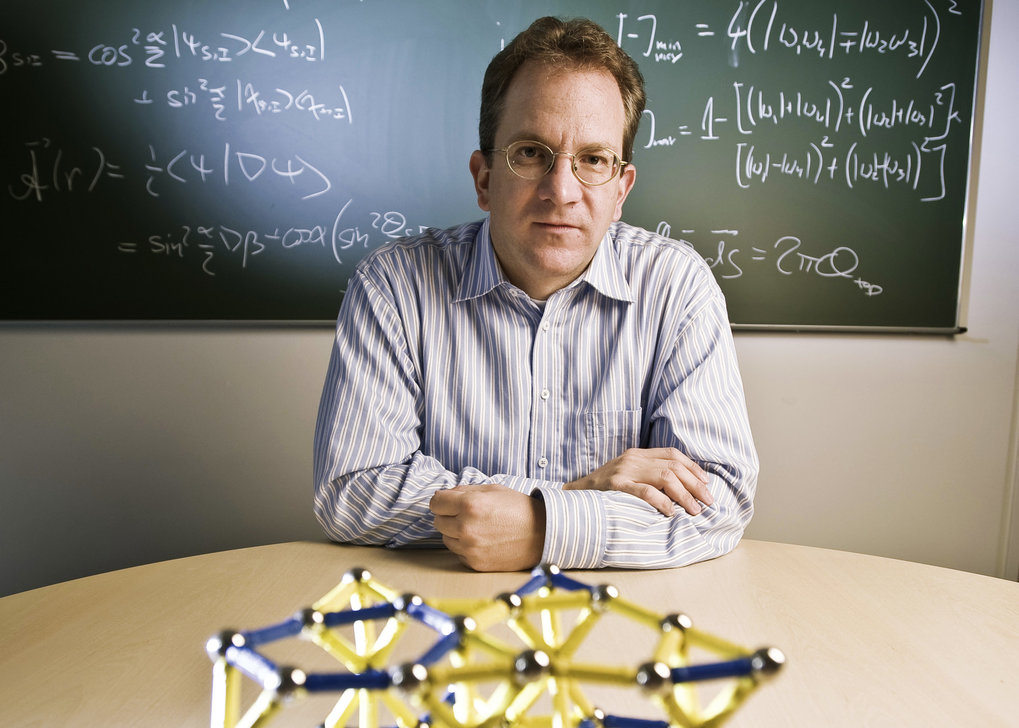
Light and shadow, good and bad, yin and yang – some opposites need each other. Physics also has an inseparable pair of opposites: the north and south poles of a magnet. To date, physicists have never been able to find reproducible evidence for the existence of a single magnetic pole – what they call “a magnetic monopole”. The majority of researchers are not surprised by this, as all known magnetic building blocks of matter, such as the electron and the neutron, always possess both north and south poles. Since our environment is made up of such particles, there should be no piece of matter that constitutes a magnetic monopole. That, at least, is the general consensus.
Against this background, the results of Roderich Moessner’s research, namely that magnetic monopoles exist in magnetic materials, seem astonishing. Moessner, a theoretical physicist and Director at the Max Planck Institute for the Physics of Complex Systems in Dresden, believes that they exist in a substance that derives its exceptionally unusual magnetic properties from its particular crystal structure: spin ice. Spins are the minute magnetic moments of the electrons in the solid, which behave like miniature bar magnets. These miniature bar magnets are arranged in spin ice in the same pattern as water molecules in ice.
In the material, magnetic north and south poles can move apart by any distance, as if freely mobile carriers of magnetic north poles and corresponding carriers of south poles existed. This is the conclusion of analyses performed by Moessner and his colleagues Claudio Castelnovo and Shivaji Sondhi at the universities of Oxford and Princeton, respectively. The researchers’ theory has attracted attention in the scientific community, not least because it has explained mysterious experimental data on spin ice.
The fact that discrete magnetic north and south poles occur in spin ice, however, does not mean that there are two new particle types, each bearing one of these magnetic poles in the same way that electrons bear a negative electric charge and protons a positive one. The textbooks of elementary particle physics do not need to be rewritten.
“Rather, the existence of magnetic monopoles is a collective phenomenon due to the interactions between the atoms and electrons of which spin ice is composed,” says Moessner. He compares the atomic teamwork in spin ice with water waves: “Individual water molecules do not exhibit waves; only a great many water molecules together are able to do so, by exerting forces on their neighbors. Waves are a simple example of a collective phenomenon.”
In solids particles exist, which are none
Waves in water and magnetic monopoles in spin ice are examples of the whole being potentially more than merely the sum of its parts. “Emergence” is the term that scientists use for the appearance of new qualities when a large number of discrete elements combine to form a new unit. An example is the combination of many individual cells to form a multicellular living organism. Solid-state physicists know of numerous emergent phenomena, such as sound waves in crystals. Many of these collective phenomena do not constitute elementary particles in the usual sense; like the discrete magnetic poles in spin ice, however, they have certain properties in common with such particles. For this reason, they are also referred to as “quasiparticles”.
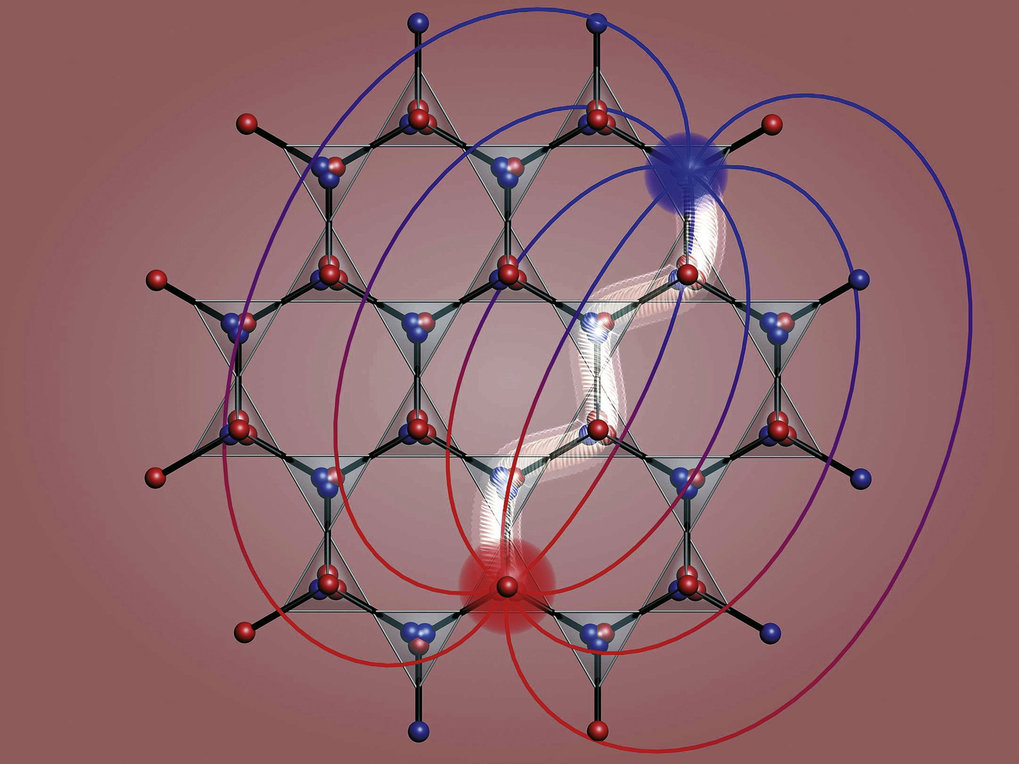
The magnetic monopoles are a class of quasiparticles that particularly fascinate physicists, while at the same time presenting them with major puzzles. “Quasiparticles exist that appear to be fragments of elementary particles that are normally considered to be indivisible,” says Moessner. The electron, for example, is defined by, among other things, two properties: its negative elementary charge and its magnetic moment of a certain strength. These two properties appear as inseparable as the two sides of a coin.
In solids, however, quasiparticles can in principle occur that, although they possess the magnetic moment of an electron, carry no charge (or vice-versa). In other words, they can be compared to one-sided coins. Against this background, it no longer seems absurd for spin ice to contain magnetic monopoles.
Solids are something different to the sum of their particles
The deconstruction of the elementary particles in the solid does not stop here: at very low temperatures and in strong external magnetic fields, quasiparticles can even occur at interfaces between two parts of the solid that bear a fraction – for example a third – of the elementary charge that is recognized as being indivisible.
In complex physical systems, with their many billions of interacting atomic nuclei and electrons, laws therefore apply that cannot be derived directly from the fundamental formulae of elementary particle physics. Such findings suggest that some materials can be regarded, in a sense, as miniature worlds that effectively create their own laws of nature.
The Dresden-based scientist is not the only one with this view. As early as 1972, American physicist Philip W. Anderson warned in the journal Science of the consequences of the reductionist research philosophy then prevailing. This philosophy assumes that things can be understood by being broken down to their smallest constituent parts. This is the route that particle physicists were taking at the time.
Anderson countered that reductionism would never be able to deliver a complete explanation of macroscopic phenomena, such as solid-state materials, plants, human beings or the universe.
He concluded his paper by saying: “The whole is not only more than, but also very different from, the sum of its parts.” In order to understand the behavior of large and complex conglomerates of elementary particles, research must be conducted that is no less fundamental than the study of the smallest particles themselves.
A hidden magnetic order gives rise to monopoles
The researchers in Roderich Moessner’s group have attempted to discover as yet unknown principles according to which complex physical systems organize in space and time. One object of their research is topological quantum liquids. In a quantum liquid, matter is present in a special state that exists in addition to the solid, liquid and gaseous phases. An example is the Bose-Einstein condensate, in which, at very low temperatures, small clouds of atoms enter a common quantum state. A quantum liquid is topological when certain of its properties are determined by its geometry. “These topological quantum liquids are currently playing an important role in the research into quantum computers,” says Moessner.
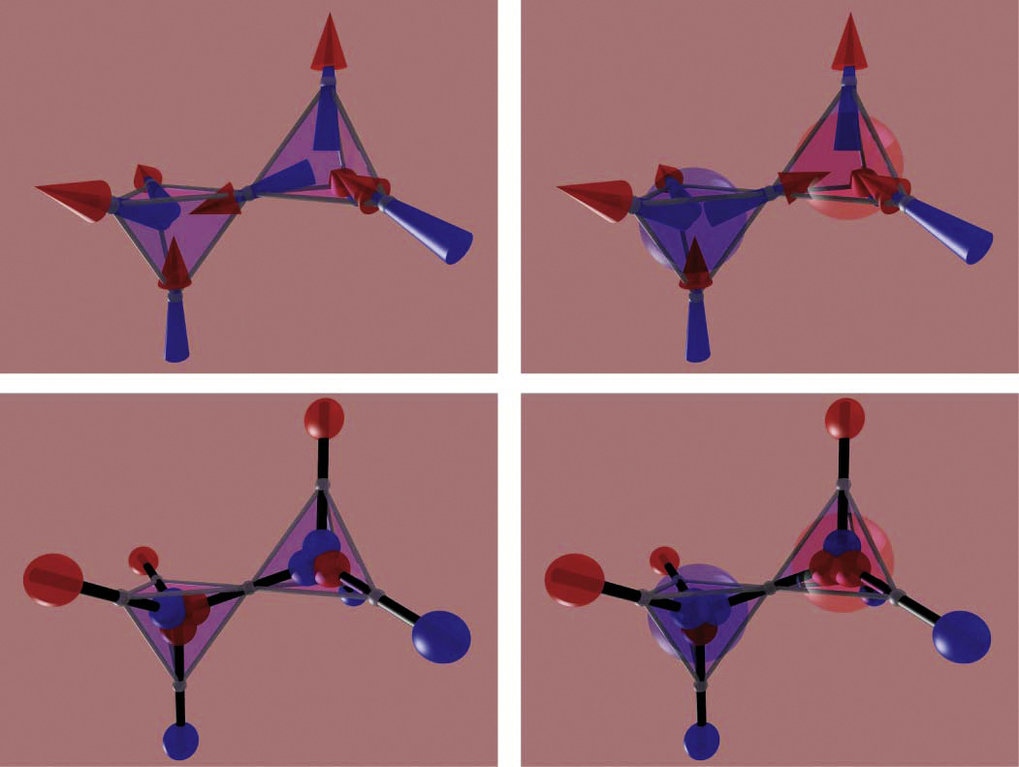
The hidden organizing principles are, in a sense, the laws of nature of complex systems, and thus the foundation of new insights. Roderich Moessner and his colleagues only predicted the appearance of the magnetic monopoles after they had observed a new form of order in spin ice.
This new type of order concerns the magnetism in the material. The magnetic order of spin ice is much less evident than, for example, that in ferromagnetic metals, which can be permanently magnetized. “In ferromagnets, the magnetic moments of all atoms tend to be aligned,” says Moessner. The arrangement of the moments can be compared to a picket fence. “To see this order, it would be sufficient to observe the alignment of the moments of a few discrete atoms,” he explains. We would then discover that most of the magnetic moments point in a certain direction. This order is local.
“In spin ice, by contrast, there is no local order,” says Moessner. But the fact that spin ice is not simply random becomes evident only when all magnetic moments in a larger region are observed. “First, however, we must consider the individual tetrahedra of which spin ice is composed,” says Moessner. Each tetrahedron is subject to the ice rule, which dictates that the magnetic moments at two of its corners have one orientation, the opposite to that of the moments at the other two corners. This permits six combinations for the orientation of the four magnetic moments at the corners of the tetrahedron, and thus six different total magnetic moments. The magnetic moments of multiple tetrahedra combine to form the magnetization of a region under consideration. Even when the ice rule is observed, different regions in spin ice may vary widely in their magnetization.
“In spin ice, one revealing quantity is the magnetization of lattice planes that run through the crystal like floors through a building,” says Moessner. The magnetization of these planes turns out to be the same as long as the ice rule is observed.
“This shows that a certain order is still present,” he says. And only where an order exists can it be violated. A magnetic monopole is nothing other than such an infringement. Initially, Roderich Moessner’s interest lay not in the magnetic monopole, but in the departure from such an order. He considered how the magnetization of the entire crystal changes when an external magnetic field acts on spin ice in the laboratory.
Exotic types of order as guiding theme
“The magnetization can change only when the ice rules are violated at certain points in the crystal. Magnetic defects are then formed at these points,” explains Moessner. These defects move through the crystal in the direction of the magnetic field, and transport the change in magnetization from one plane to another. When he analyzed the properties of these defects, the physicist noticed that they were magnetic monopoles: they behave in an analogous way to electrical charges.
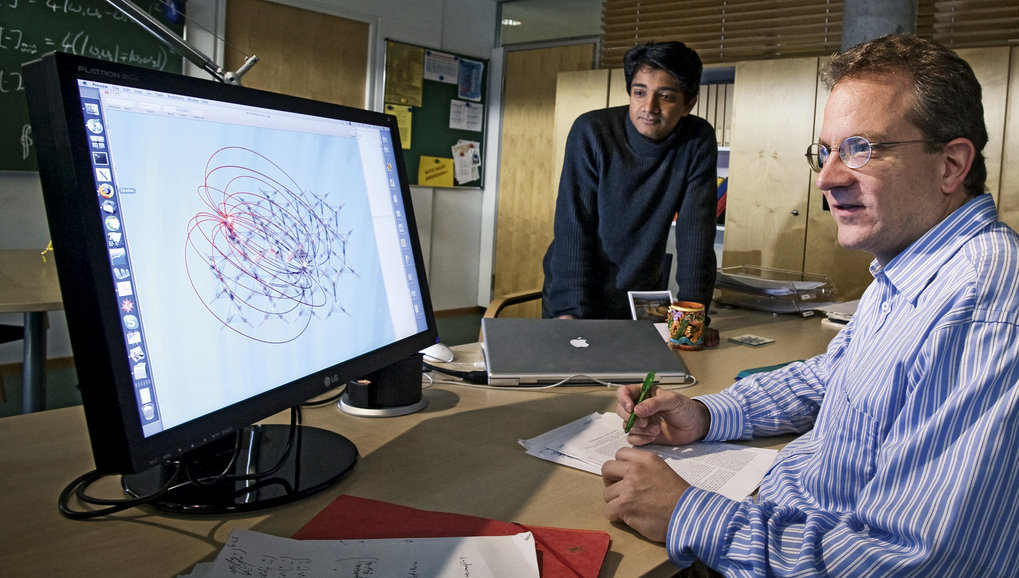
The special properties of spin ice were not discovered by chance. The search for hidden and exotic types of order has been a recurring theme throughout Moessner’s research career. While working on his doctoral studies, he studied two-dimensional electron gases, and predicted electronic states that were found to lead to orders resembling those in liquid crystals. In liquid crystals, molecules align along one spatial direction; otherwise, they remain random. The existence of these electronic states was later verified experimentally.
Moessner subsequently conducted research in Hamilton (Canada) and at Princeton University in the US. There, he showed that – surprisingly simple – microscopic models exist in which the elementary charge can be separated from the magnetic moment of an electron – that is, the spin. To understand how this happens, one can imagine that the electrons in the solid move on a lattice. The negative charges of the electrons are compensated by the positively charged ions.
In the lattice planes, each electron is surrounded by its neighbors. It pairs off with one of these neighbors such that the spins of the two electrons – their magnetic moments – cancel. However, these pairings are ephemeral. Each electron continually changes partners, with the result that the entire arrangement of bonds is in constant motion.
“If a single electron is removed from the lattice, a hole, a positive charge, is left behind, and next to it, an unpaired electron,” explains Moessner. The remaining solitary electron is evident only from its spin; against the positive background, its negative charge goes unnoticed, as before. The electron need not remain alone, however; it can capture a partner from an adjacent spin pair. The unpaired spin, or the magnetic moment, thus migrates through the lattice, without the positive charge following it.
Experiments are prepared to demonstrate magnetic monopoles
In his book A Different Universe: Reinventing Physics from the Bottom Down, published in 2005, physicist and Nobel laureate Robert B. Laughlin predicts new discoveries of many such organizing principles and laws of nature in complex systems. According to Laughlin, we are still unaware of the great majority of organizing principles. So the physicists in Roderich Moessner’s group still have much work to do.
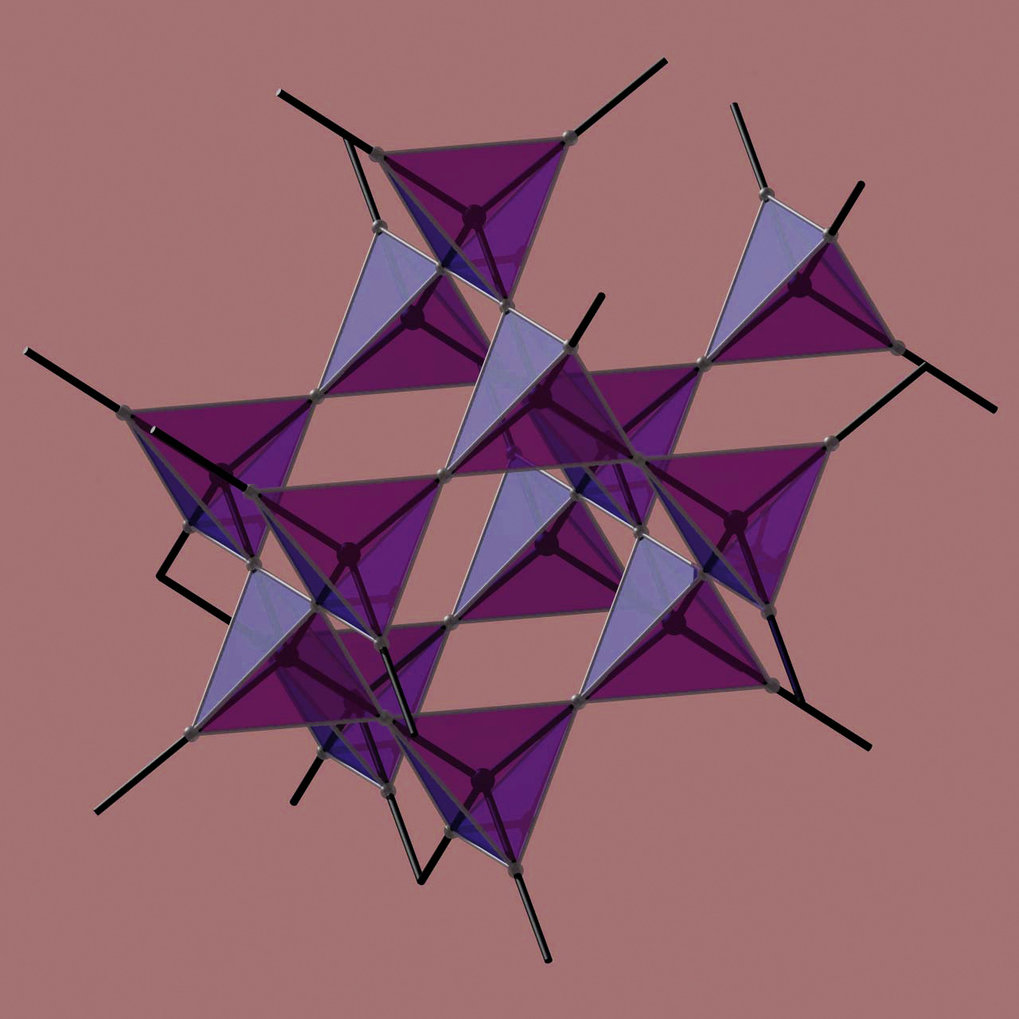
Theoretical research into complex systems fascinates him for two reasons. “For one thing, we work in small groups, which gives us a high degree of flexibility and variety,” says Moessner. The second reason is probably more important: “Particle and astrophysicists have one large universe. We have many small quasiuniverses,” he says jokingly. “For us, every material is a potentially new type of complex system.” The variety within solid-state physics, says Moessner, derives from the countless possible combinations of the elements in the periodic table. So there are many new phenomena yet to be discovered.
The results of the scientists’ research into miniature quasiuniverses should not remain purely theoretical. Experiments on the monopole hypothesis are now on the agenda. Preparations for these are underway at several locations. “Whatever the results of the experiments, I am confident that they will teach us something important,” says the physicist. He does not yet envisage direct technical applications of the magnetic monopoles. However, the good news, says Moessner, is that it will hopefully be possible to create magnetic monopoles should they be needed for a specific application. “This demonstrates the level of control that we are now able to exercise in materials physics and the physics of complex systems.”
GLOSSARY
Spin Ice
Spin ice forms in a crystal lattice of tetrahedra that are joined at their corners. It is composed of the elements holmium or dysprosium, titanium and oxygen. Spin ice owes its name to a similarity with water ice. The latter consists of oxygen and hydrogen atoms. Each oxygen atom has four neighboring hydrogen atoms. Two of these – the two with which the oxygen atom forms a water molecule – are closer to the oxygen than the other two. Experts call this the ice rule. Spin ice has a similar local arrangement of the magnetic moments of the spins located at each corner of the tetrahedron. Magnetic moments resemble minute bar magnets, each with a north and a south pole. Like the needle of a compass, the magnetic moments are pivoted at each of the four corners of the tetrahedra that make up spin ice. In the lowest-energy and thus preferred state of spin ice, two north poles in each tetrahedron point to its center, and two away from it – a magnetic version of the ice rule.