Intercalation and doping of expitaxial graphene
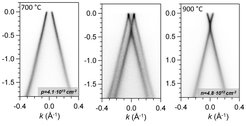
The growth and investigation of graphene (a single layer of carbon atoms) on SiC possesses several major advantages compared to graphene on metallic surfaces. Due to its band gap of around 3 eV SiC is an ideal candidate to investigate electronic properties of graphene. Therefore, no transfer of graphene onto semiconducting or insulating substrates is necessary, which often deteriorates the properties of graphene and is not scalable for mass production. However, after the growth of one carbon layer on SiC by silicon sublimation at high temperature, some of the carbon atoms are still bond to the silicon atoms of the surface and therefore the carbon layer does not show graphene’s typical Dirac like band dispersion. These bonds between Si and the carbon layer can be broken by the intercalation of foreign atomic species, so that the carbon layer is decoupled and the π band system develops its typical conical dispersion. In addition, the carrier density of the epitaxial graphene can be precisely controlled. A milestone in this type of modification of epitaxial graphene (EG) was the preparation of quasi-free standing monolayer graphene (QFMLG) on SiC(0001) by means of atmospheric hydrogen intercalation (Riedl 2009). Intercalation of other elements allows us to functionalize the π band structure, so that p- and n-doped phases could be retrieved by controlled Ge (Emtsev 2011) or Au intercalation (Gierz 2010). Cu intercalation imposes a superperiodic potential onto the graphene layer leading to the appearance of so-called mini-Dirac cones (Forti 2016). New intercalation approaches by implantation and segregation where invented for Bi (Stoer 2016) and also Sb. Besides the generation of quasi-free standing decoupled graphene strongly doped graphene (Yb, Gd) or even 2D metallic bands in-between graphene and the SiC surface (Au, Ag) can be created.
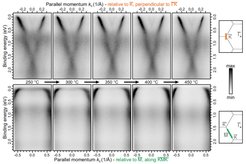
ARPES on Yb intercalated graphene for different annealing temperatures (Rosenzweig 2019).
Yet, also without intercalation, the deposition of molecular layers or alkali metals can be applied to tailor the charge carrier density in epitaxial graphene. F4-TCNQ was used to obtain charge neutral graphene (Coletti 2010). Further, It could also be demonstrated that Li could potentially induce a superconducting state by an enhanced electron-phonon coupling that leads to a deviation from the Fermi distribution within the cut-off of the topmost valance bands, i.e., evidence for a superconducting gap was found (Ludbrook 2015).
Publications:
- P. Rosenzweig, U. Starke, Phys. Rev. B 101, 201407(R) (2020). Large-area synthesis of a semiconducting silver monolayer via intercalation of epitaxial graphene.
- S. Forti, S. Link, A. Stöhr, Y. Niu, A. A. Zakharov, C. Coletti, U. Starke, Nat. Commun. 11, 2236 (2020). Semiconductor to metal transition in two-dimensional gold and its van der Waals heterostack with graphene.
- P. Rosenzweig, H. Karakachian, S. Link, K. Küster, U. Starke, Phys. Rev. B 100, 035445 (2019). Tuning the doping level of graphene in the vicinity of the Van Hove singularity via ytterbium intercalation.
- S. Link, S. Forti, A. Stöhr, K. Küster, M. Rösner, D. Hirschmeier, C. Chen, J. Avila, M. C. Asensio, A. A. Zakharov, T. O. Wehling, A. I. Lichtenstein, M. I. Katsnelson, U. Starke, Phys. Rev. B 100, 121407(R) (2019). Introducing strong correlation effects into graphene by gadolinium.
- A. Stöhr, S. Forti, S. Link, A.A. Zakharov, K. Kern, U. Starke, and H.M. Benia, Phys. Rev. B 94, 085431 (2016). Intercalation of graphene on SiC(0001) via ion implantation.
- S. Forti, A. Stöhr, A. A. Zakharov, C. Coletti, K. V. Emtsev, and U. Starke, 2D Mater. 3, 035003 (2016). Mini-Dirac Cones in the Band Structure of a Copper Intercalated Epitaxial Graphene Superlattice.
- B.M. Ludbrook, G.Levy, P. Nigge, M. Zonno, M. Schneider, D.J. Dvorak, C.N. Veenstra, S. Zhdanovich, D. Wong, P. Dosanjh, C. Straßer, A. Stöhr, S. Forti, C.R. Ast, U. Starke, and A. Damascelli, Proc. Nat. Acad. Soc. 112, 11795 (2015). Evidence for superconductivity in Li-decorated monolayer graphene..
- K. Emtsev, A.A. Zakharov, C. Coletti, S. Forti, and U. Starke, Phys. Rev. B 84, 125423 (2011). Ambipolar doping in quasi-free epitaxial graphene on SiC(0001) controlled by Ge intercalation.
- I. Gierz, T. Suzuki, R.T. Weitz, D.S. Lee, B. Krauss, C. Riedl, U. Starke, H. Hochst, J.H. Smet, C.R. Ast, and K. Kern, Phys. Rev. B 81, 235408 (2010). Electronic decoupling of an epitaxial graphene monolayer by gold intercalation.
- C. Riedl, C. Coletti, T. Iwasaki, A.A. Zakharov, and U. Starke, Phys. Rev. Lett. 103, 246804 (2009). Quasi-free standing epitaxial graphene on SiC obtained by hydrogen intercalation.