Electron Tunneling at High-Temperatures
The motion and storage of ions provide key functionalities in solid-state devices. Analytical tools are therefore sought that allow the behavior of ions in solids, in particular along interfaces, to be monitored and analyzed with high spatial resolution and in real time. In principle, inelastic electron tunneling spectroscopy (IETS) offers these capabilities [1-4].
However, while the ion dynamics well above room temperature is of key interest for solid-state ionics, IETS has been mostly limited to the liquid helium temperatures due to the thermal smearing of the electron distribution in metal contacts at high-temperatures [2].
As reported in Advanced Materials 2007299 (2021), we have broken this limit arising from the thermal softening of the tunnel electrodes' Fermi–Dirac distributions. This breakthrough now allows for the use of IETS for high-resolution analysis of ion populations well above room temperature.
To experimentally investigate ionic diffusion along interfaces [5] and explore the potential of IETS to study ionic species at high-temperatures, we have used the same BaZrO3-based heterostructures as proton conductors and electron tunnel barriers (Figure 1).
By analyzing O–H bond vibrations, the existence of protons in the tunnel barriers is confirmed (Figure 2). The tunnel junctions yield high-resolution IETS spectra of diffused protons along interfaces in BaZrO3–BaYOx-based tunnel barriers up to at least 400 K (Figure 3). We demonstrate IETS spectral resolution that is a factor of nine better than the established theoretical limit. With these advances, IETS constitutes a viable high-resolution analytical tool for further nanoionic and nanoelectronic studies at high-temperatures in nanostructured tunnel junctions (Figure 4).
This research was done in collaboration with Joachim Maier of the Department for Physical Chemistry of Solids, and Yi Wang and Peter A. van Aken of the StEM group.
References
[1] R. C. Jaklevic and J. Lambe, Physical Review Letters 17, 1139 (1966).
[2] J. Lambe and R. C. Jaklevic, Physical Review 165, 821 (1968).
[3] J. W. Reiner et al., Advanced Materials 22, 2962 (2010).
[4] M. A. Reed, Materials Today 11, 46 (2008).
[5] P. Ngabonziza, R. Merkle, Y. Wang, Peter van Aken, T. S. Bjørheim, J. Maier, and J. Mannhart, Advanced Energy Materials, 1600157 (2020).
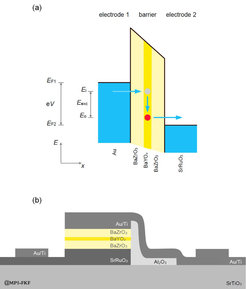
(a) Schematic illustration of an inelastic electron tunnelling process for device with proton-conducting BaZrO3–BaYOx–BaZrO3 barriers. The embedded BaYOx layer is a diffusion channel for protons. The energy spectrum of the vibrational modes caused by the protons present in the BaYOx layer can be derived by measuring Eexc, which is a characteristic property of the particle of interest. (b) A corresponding cross section layout of a SrTiO3–SrRuO3–BaZrO3–BaYOx–BaZrO3–Au tunnel junction device.
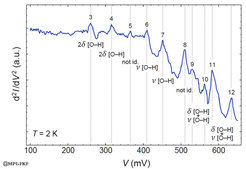
High-resolution IETS spectrum displaying the assigned O–H bond vibrations.
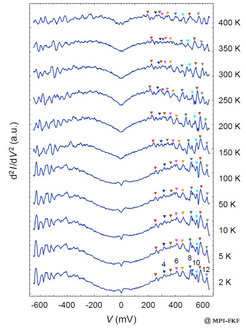
IETS spectra measured at a range of temperatures. The low-energy IETS features (230 meV) are well resolved and traced up to 400 K.
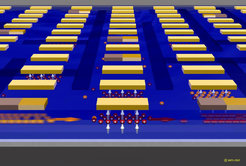
Illustration of a nanoionic device with tunnel sensors monitoring the diffusion of two species of ions (orange and red spheres) along ion-conducting passages (bright blue). Ions diffusing in plane are detected by transversely tunneling electrons (silverish arrows). The ions induce inelastic electron tunneling, which causes ion-specific features in the electron tunneling characteristic. These can be monitored and spatially resolved in real time. The bicolored brown bars depict electrodes.